Membrane dialysis is a simple and widely used biochemical technique during protein purification to separate small molecular weight substances. In the process of isolating an industrially useful acid protease from locally available Aspergillus species, ammonium sulphate was used as salting-out agent in the very initial step of protein purification. While subjecting the ammonium sulphate precipitated-protease enriched sample for dialysis, we repeatedly found irregular perforations in the dialysis tubing. Careful analysis of the protease source suggested that, cellulase present in the protease enriched sample of Aspergillus species was responsible for this pore-forming act in the membrane. A simple gel filtration on Biogel-P100 (polyacrylamide beads) effectively separated ammonium sulphate from protease and cellulase, thereby avoiding dialysis. These studies provide one of the limitations of dialysis technique and suggest not to use cellulose- based dialysis tubing while dealing with samples containing cellulases.
Protein purification is vital to understand the structure and functions of any protein. The method of protein purification generally involves multiple chromatographic separation steps [1]. While purifying proteins from natural sources, concentrating the enzyme of our interest is a primary requisite. Commonly used concentrating methods include ultra filtration [2], reverse dialysis using sucrose [3], precipitation using chilled solvents (4°C to -20°C) [4,5] and salting out using divalent cationic salts [6]. Of these, salting out method of protein precipitation is commonly used. The most sought salt to perform salting out is ammonium sulphate [7]. However, it is essential to remove ammonium sulphate for subsequent purification of proteins, as it interferes with many of the further purification steps and protein quantification [8,9]. However, these conventional protein isolation methods are quite time consuming and involve multiple steps. Recently an Aqueous Two-Phase Floatation (ATPF) or Liquid Biphasic Floatation (LBF) technique has also been developed and successfully implemented in several instances [10,11].
Proteases derived from lower organisms have enormous industrial applications and researchers are on a quest for newer sources of proteases with high catalytic turnover and stability. In the process of searching for an industrially useful acid protease, we came across a strain of Aspergillus niger that produces thermostable acid protease. We initially enriched this enzyme using ammonium sulphate salting out method. The protease was salted out with 50% ammonium sulphate. To remove ammonium sulphate for the next step, we employed dialysis tubing with a molecular weight cut off 10KDa. We found dialysis tubing undergoing multiple, irregular perforations in the membrane, resulting in loss of the sample. We initially suspected a manufacturer’s defect in dialysis tubing, but that was not the case. Upon careful analysis, we found that perforation was due to co-eluting cellulase(s) acting on dialysis tubing. We then successfully employed biogel-P100 (gel filtration matrix made out of polyacrylamide beads) to remove ammonium sulphate. We believe that these findings offer a precautionary message both to students and researchers alike while dealing with fungal enzymes.
Materials
Aspergillus niger culture was from Kaypeeyes biotech Pvt Ltd, Hebbal Industrial Area, Mysuru, Karnataka, India. Carboxymethylcellulose (CM-cellulose) (Sodium salt) and dialysis tubing (cellulose membrane) were obtained from SigmaAldrich, St. Louis, Missouri, USA; Haemoglobin was from MP Biomedicals, Santa Ana, California, USA;Biogel-P-100 was from Biorad, Hercules, California, USA; Spin columns were procured from GE healthcare, Little Chalfont, UK; Trichloroacetic Acid (TCA), Dinitrosalicylic Acid (DNS) and Nessler’s reagent were obtained from Lobachemie, Mumbai, Maharashtra, India.
Methods
Production of acid protease from A.niger: A. niger was grown by solid state fermentation using wheat bran as substrate. To 100 g of wheat bran, 60 mL of 0.2 N HCl with trace minerals (70 mg each of CuSO4, ZnSO4 and FeSO4 per 100 mL of 0.2NHCl) was added. Sterile wheat bran was inoculated with A. nigerspore suspension and incubated at 30°C for 7 days. The extracellular acid protease present in the fermented material was extracted in 0.1 M NaCl and recovered by centrifugation at 7000 g for 10 min at 4°C and filtered through Whatman filter paper no. 1. The clear supernatant was used as enzyme source.
Assay of acid protease: Acid protease was assayed as described by [4] with slight modifications. Briefly, 2% haemoglobin (acid denatured) prepared in 0.1 M acetate buffer pH 4.0 was used as substrate. To this, 0.4 mL (0.5-5µg) enzyme was added and incubated at 60°C for 10 min. The reaction was arrested by the addition of 2 mL of 5% TCA and the tubes were incubated at room temperature for 20 min. After 20 min, the reaction mixture was filtered through Whatman filter paper no.1 and the absorbance of the supernatant containing released tyrosine was measured at 280 nm. The amount of tyrosine released was calculated using a tyrosine standard (0-60 µg/mL). One unit of protease activity is defined as the amount of enzyme required to liberate 1µg of tyrosine per minute, under standard assay conditions.
Assay of cellulase: Cellulase assay was carried out as described by Wood and Bhat [12] with slight modifications. In brief, 0.1 mL (50-150µg) enzyme was added to 0.9 mL of 1% CM-cellulose prepared in 0.1 M acetate buffer pH 5.0. The enzyme reaction was carried out at 40°C for 120 min. The reaction was terminated by the addition of 1 mL of DNS reagent. The tubes were boiled in boiling water bath for 10 min, cooled and added with 8 mL of distilled water. The absorbance was read at 540 nm and the amount of glucose released was calculated using a glucose standard (0-1000 µg/mL). One unit of cellulase activity is defined as the amount of enzyme that liberates 1µmol of reducing sugar (expressed as glucose) per minute, under standard assay conditions.
Ammonium sulphate fractionation of acid protease: To the culture supernatant, ammonium sulphate was added to 30% saturation and then subsequently to 50% and 60%, with constant stirring at 4°C for 6 h. The precipitate was collected by centrifugation at 7000 g for 30 min at 4°C (in some experiments an aliquot from each fractionation was kept aside for assays of protease and cellulase).
Dialysis: 50% ammonium sulphate pellet showing the highest specific activity was re-suspended in 50 mM acetate buffer pH 5.0 containing 0.1 M NaCl and dialyzed against same buffer.
Biogel-P100 chromatography: 50% ammonium sulphate pellet showing the highest specific activity for protease was resuspended in 50 mM acetate buffer pH 5.0 containing 0.1 M NaCl. This sample (2ml containing 100mg protein) was loaded on to a 1.5cm × 78cm column packed with 137ml of Biogel P100 polyacrylamide beads, equilibrated with 50 mM acetate buffer pH 5.0 containing 0.1 M NaCl and eluted with the same buffer. Fractions were collected at a flow rate of 20ml/h and monitored for absorbance at 280nm. Each fraction was assessed for the presence of protease, cellulase and ammonia (see below).
Protein estimation: Protein estimation was carried out as described by [13] and Biuret’s method [14]. A) Lowry’s method: This method involves two reactions leading to color complex formation. In first reaction copper [II] reacts with the peptide bond in the protein under alkaline conditions, resulting in their reduction to cuprous [I] ions. The subsequent reaction involves the reduction of phosphomolybdicphosphotungstic acid to heteropolymolybdenum blue by the copper-catalyzed oxidation of aromatic acids. The blue complex formed is measured at 660 nm. The concentration of protein present in the sample is calculated using standard graph constructed with BSA as standard (15- 75 µg/mL). B) Biuret method: Under alkaline condition copper [II] ions reacts with peptide bond and forms mauve-colored coordination complex, which is measured at 540 nm. Amount of protein present is calculated using standard graph constructed with BSA (1-5 mg/mL).
Quantification of ammonia: Ammonia quantification was done using Nessler’s reagent [15]. Each fraction from Biogel P-100 was treated with Nessler’s reagent and absorbance was read at 425 nm. The amount of ammonia was calculated using a standard (1-20µg/ml) made with ammonia solution.
Dialysis is a simple technique, yet not useful for samples containing cellulases.
The global sales of industrial enzymes account for more than 3 billion USD of which proteases constitute more than 60% [16]. These proteases have wide range of applications and are mostly derived from microbial sources [17]. Among the microbes, enzymes derived from filamentous fungi such as Aspergillusspecies have many advantages over the other microbial sources, due to low cost of production, amenable for easy downstream processing, coupled with faster growth of the fungi and secretory nature of the enzyme [18]. While screening for an industrially useful protease from Aspergillus species, we came across an acid protease from Aspergillus niger which had industrially desirable qualities such as high catalytic turnover and thermostability. Hence, we decided to purify this fungal protease by conventional protein purification techniques. Precipitating the proteins by ammonium sulphate (salting out method) is a routine and simple technique widely used during protein purification. However, subsequent removal of residual ammonium sulphate is very much essential for further purification of the enzyme. In an effort to separate ammonium sulphate from the sample, dialysis was carried out using nitrocellulose membrane. But, every time during dialysis the sample was lost, as a result of formation of pores in the membrane (Figure 1). Although we suspected manufacture’s defect initially, later we found an enzyme culprit responsible for this act. This culprit was cellulase. Accordingly, we found detectable cellulase activity in all the fractions that were also positive for proteases (Table 1). In order to separate ammonium sulphate we used spin columns (made of polyethersulfone) but this resulted in low recovery of the enzyme. Hence, we proceeded with differential ammonium sulphate fractionation, in an attempt to separate cellulase from protease. However, cellulase activity was also enriched with protease activity in all the steps of differential ammonium sulphate fractionation (Table 1). Therefore, we decided to use other chromatographic separation steps, which require prior removal of ammonium sulphate. Among the available methods, simple dialysis was the method of choice. However, we came across the problem of cellulase action on dialysis tubing. Therefore, dialysis is not always the best technique for the removal of small molecules. Cellulases degrading cellulose is not new [19], but while purifying proteases co-purifying cellulases degrading cellulose was new to us. Similar difficulty was also encountered recently by Monico et al., where dialysis was not efficient in removing EDTA [20]. Other option for us at this stage was to use non-cellulosic dialysis membrane, but then they are expensive and often available in broad cut off range and not available with all the vendors. Hence, we decided to use biogel-P100.
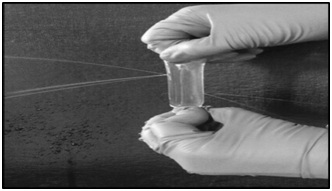
Figure 1: Perforation in dialysis tubing: The crude fungal extract was precipitated with solid ammonium sulphate (see methods). The extensive dialysis resulted in the formation of pores in dialysis tubing due to cellulase action. Note the loss of sample from dialysis tubing when squeezed downwards.
Biogel-P-100 effectively removes ammonium sulphate
Once we realized that cellulase was co-purifying with protease of our interest, carrying out dialysis using cellulose based membrane would be a futile exercise. Therefore, we attempted to remove ammonium sulphate by molecular sieving. A 50% ammonium sulphate pellet showed significant protease and cellulase activity (Table 1). This fraction was loaded on to a biogel P100 column. Loaded proteins were eluted (fraction number 20-42) in the first peak (Figures 2A and 2B), while the ammonium sulphate (fraction number 52-69) eluted later (Figures 2C and 2D). We confirmed elution of ammonium sulphate by both Nessler’s reagent and measuring the conductance. The fractions containing proteins (fractions 20-42) showed no reactivity to Nessler’s reagent while fractions containing ammonium sulphate reacted (fractions 52-69) to Nessler’s reagent and showed conductance (Figures 2C and 2D).
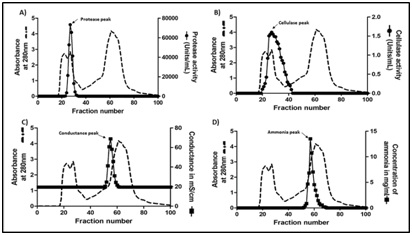
Figure 2: Molecular sieving using biogel-P100. 2 ml of 50% ammonium sulphate pellet containing 100 mg protein was subjected to molecular sieving using biogel-P100 previously equilibrated with 50 mM acetate buffer pH 5.0 containing 0.1 M NaCl and the fractions were monitored for their absorption at 280nm. Each fraction was assayed for A) protease activity B) cellulase activity C) conductance D) ammonia by Nessler’s reagent. Notice the co-elution of both protease (fraction 23-33, A) and celluase (fraction 20-42, B). Ammonium sulphate elutes from fraction 52-69 as confirmed by both conductance and positive reaction to Nessler’s reagent. Data represents typical elution profile in more than 3 separate experiments. Notice the absorbance at 280nm seen in all the panels (---) corresponds to proteins (fraction 20-42) and uncharacterised coloured substances (fractions≈ 44-80).
Sample
|
Volume mL
|
Protein mg/ml
|
Protease
|
Cellulase
|
|
|
Activity U/mL
|
Specific activity U/mg
|
Activity U/mL
|
Specific activity U/mg
|
|
|
Crude extract
|
47
|
15.4
|
3835.8
|
249
|
2.64
|
0.171
|
|
Ammonium sulphate 30% supernatant
|
50
|
13
|
4319.9
|
332.2
|
1.49
|
0.114
|
|
Ammonium sulphate 30% pellet
|
4
|
50
|
4748.5
|
94.96
|
11.35
|
0.227
|
|
Ammonium sulphate 50% supernatant
|
49
|
8
|
95.2
|
11.9
|
-
|
-
|
|
Ammonium sulphate 50% pellet
|
4
|
53.8
|
37281.1
|
703.4
|
16.9
|
0.314
|
|
Ammonium sulphate 60% supernatant
|
46.5
|
6
|
12.87
|
6.4
|
-
|
-
|
|
Ammonium sulphate 60% pellet
|
0.8
|
16
|
5003.7
|
312.6
|
0.78
|
0.048
|
|
Table 1: Ammonium sulphate fractionation of protease. Calculated amount of ammonium sulphate was added to culture supernatant to achieve 30-60% saturation. Protease and celluase activity were measured as described under methods. Both protease and cellulase co-precipitated at each saturation step and highest specific activity was seen in 50% pellet.
Although dialyzing out ammonium sulphate is widely used during protein purification, it is not recommended specially for the samples containing cellulases. Molecular sieving using biogel-P100 instead of dialysis effectively removes ammonium sulphate from rest of the sample. As almost all fungi are known to have cellulases, along with other enzymes, commonly available dialysis membranes are likely to be degraded by the cellulase enzyme present in the fungal preparation. Hence, we highly recommend not using cellulose-based dialysis tubing while working with fungal enzymes.
Authors greatly acknowledge the financial support and Aspergillus culture from Kaypeeyes biotech Pvt. Ltd. Mysore, India. GKM thanks Institute of excellence, University of Mysore, UGC-Major Research Project, Vision Group of Science & Technology (VGST), Government of Karnataka and UGC-Special Assistance Program (UGC-SAP). The funders had no role in study design, data collection and analysis, decision to publish, or preparation of the manuscript. Authors of this manuscript declare no conflict of interest.